Economics of Battery Energy Storage Systems
Batteries form a cornerstone of our modern society, powering our portable gadgets throughout the day before we can charge them to begin anew. Batteries and energy storage at large represent an opportunity to match energy demand with supply to ensure our needs for energy are met through the time we desire it to. This article is focused on the storage of electrical energy to match our demand. Electrical energy storage requires conversion to another form of energy such as thermal, chemical, mechanical, gravitational, or electro-static forms. These types of storage are typically used for different practical use cases depending on need.
The growing requirement for storage on our electricity grids has led to the interesting rise of energy storage as an asset class. An asset that has a unique revenue profile compared to the bread and butter of energy generation assets such as solar and wind. Given the uniqueness of energy storage as an asset class, I thought it would be an interesting topic to dive into and understand the niche mechanics of these systems. Given I spent the first year of my career working in long duration energy storage, I thought that understanding shorter term energy storage systems would be a valuable research topic. This article is focused on utility-scale BESS assets.
Science of Batteries
Before jumping into the dollars and cents of it all, I want to provide an extremely high level overview on the science behind batteries as understanding a little about the science goes a long way to understand the economics. Batteries have different compositions based on the composition of the anode, cathode, and electrolyte. Based on these compositions, each combination will lead to different design and operational parameters to meet different needs such as a higher energy density for electric vehicles. At its essence, a battery stores electrical energy in the form of chemical energy. The chemical reaction in a battery involves the flow of electrons from anode to cathode.
At the anode (negative), chemical reactions cause electrons to build up resulting in an electrical difference between the anode and the cathode. Due to the imbalance, the electrons now want to flow to the cathode to get rid of the imbalance. When the circuit is closed, the electrons are able to flow to the cathode (positive). When you recharge a battery, the reverse of this reaction occurs.

Types of Batteries
As discussed above, the composition of the anode, cathode, and electrolyte determine characteristics about the battery. This section will briefly summarize the dominant forms of batteries today to provide context on different types of battery technologies.
- Lithium-ion Batteries: This is the most common type of battery and is estimated to compose 90% of the grid battery storage market. This is also the type of battery used to store energy in our cell phones and laptops. Given the prevalence of lithium-ion batteries, this article will assume that this technology is the default wherever batteries are referred to.
- Lead-Acid Batteries: These are the most mature battery technology with the first applications dating back to the 1860s. Given the length of time these batteries have been around, they now have low costs with the tradeoff of limited life cycles and energy density.
- Sodium-Sulphur Batteries: A relatively new technology, initially designed for electric vehicles, they are now a low-cost option for grid storage and renewable applications. Given the need for the high temperature for the liquid sodium aspect, these batteries can have 20% daily losses due to heat dissipation.
- Flow Batteries: The most common flow battery is the vanadium redox battery. These batteries have a very long lifecycle, low discharge losses, and moderate efficiency and costs. Compared to other batteries, these have a lower technology readiness level with few small commercial applications to date.
Growth of Batteries
While pumped hydro energy storage is currently the most widely deployed storage technology, grid-scale batteries are rapidly growing thanks to their declining costs and increased market acceptance. As of 2021, total pumped hydro energy storage installed capacity stood as ~160 GW. In contrast, total grid-scale BESS amounted to 28 GW at the end of 2022 with the US and China leading the way for deployment. The International Energy Agency expects that to reach net zero targets, the installed grid-scale BESS capacity must grow 35x from 2022 to 2030. This translates to an average of ~120 GW per year between 2022 and 2030 to hit these ambitious targets.
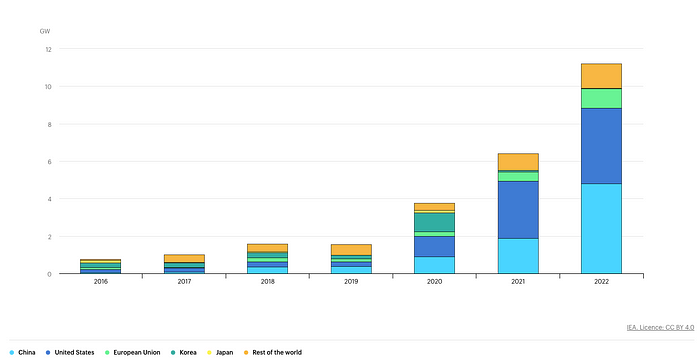
Batteries are experiencing rapid growth as their value is being recognized as essential as the grid integrates more sources of variable renewable energy. Energy storage enables the smoothing of the fluctuating output that solar and wind create that would otherwise negatively impact the grid by harming the frequency balance. Given solar and wind can lead to grid instability, the short charge-and-discharge cycles of batteries are able to respond to these fluctuations to ensure stability. Further, batteries are able to integrate renewables by shifting supply to demand and help avoid curtailment of these resources. The avoidance of curtailment requires batteries with longer duration of storage.
Economics of BESS
We have a grasp on the science and types of batteries available, it is time to dive into the economics of these assets. Just like some other renewable energy assets, BESS assets earn revenue, incur costs to operate, have an upfront capital cost, and degrade. The intricacies come into play as we dive deeper into each financial element. This section of the article will go further in depth on each of these items as we look to understand how these assets produce cash flow. The cost economics of batteries can be quantified using a levelized cost of storage (LCOS) approach to account for all financial and technical considerations affecting the total lifetime dynamics of these assets.
Simply put, the LCOS of BESS assets are the capital costs, plus cumulative operation costs, plus cumulative charging costs, plus cumulative end of life costs. These costs are divided by the total electricity discharged by the system. All elements are discounted at the appropriate discount rate with the exception of the capital costs given the capital costs are incurred at the beginning of the analysis. The end result is a $/hourly unit figure such as $/MWh or $/kWh.
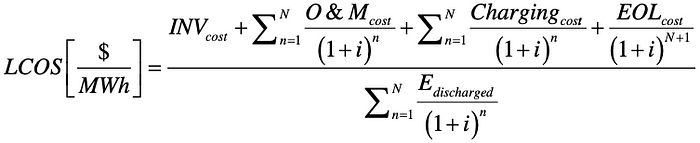
Revenue
Before diving into costs of these assets, understanding what they can earn in terms of revenue is key to understand the operating profile and how these assets function on the electricity grid. Noting that revenue is actually highly dependent on the electricity grid upon which the asset connects to. There are various revenue streams that an asset can earn depending on the market rules. For that reason, I will provide a list of the key revenue streams available to batteries. It is important to note that BESS assets can earn multiple revenue streams. In fact, operating in such a way to maximize benefits to the asset operator is called value stacking and is key to stacking services and unlocking the maximum financial impact possible.
1 — Capacity Payments (Resource Adequacy)
These are payments that are made to ensure that resources such as BESS assets are available for a certain period of time. These are payments received simply for standing by and being ready to discharge regardless if an asset is called upon or not. The payments act as financial incentive for the assets to maintain a level of capacity at all times, ensuring that there is enough electricity to meet peak demand and prevent power shortages.
The payments are administered through the capacity market, a mechanism used to procure and compensate power generation resources for the provision of power during periods in which it is required. These markets are typically regulated and operated by Independent System Operators (ISOs) and Regional Transmission Organizations (RTOs) in which capacity markets are available. The ISOs and RTOs ensure the reliability and adequacy of the electricity supply. A capacity market works by having assets bid to offer a certain amount of capacity to the grid over a specific period of time. The bids are evaluated based on several factors including availability, capability, and cost. Successful bidders receive the payments as incentives for the bidders to be available when called upon.
2 — Energy Arbitrage
Energy arbitrage is where batteries become very unique assets. Arbitrage is the act of purchasing an asset low and selling the same asset for a higher price. Batteries have two cycles, charge and discharge. Or put another way, the purchasing and selling of electricity to power the BESS asset. As such, BESS assets can earn revenue by purchasing electricity to charge the system during periods of low electricity prices and by selling electricity to the grid when prices are high. The spread between the discharge revenue and the charge costs is the net revenue that is earned for the period.
Energy arbitrage revenues are considered riskier as they require active management that creates volatility and less forecast visibility into the expected revenues versus a contracted revenue stream. Additionally, a quirk of arbitraging energy with batteries is that batteries actually degrade over time, reducing the effective capacity of these assets. This concept of degradation has a separate paragraph below with further detail.

3 — Ancillary Services
Ancillary services are functions of assets on the grid that enable the grid operators to maintain a reliable electricity system. These services ensure the proper flow of electricity, help the system recover after a black out / brown out, and balance supply and demand. As further renewables are connected to the grid, ancillary services are becoming increasingly important to manage increased variability and uncertainty. They are required services to ensure the system operates within acceptable frequency and voltage levels and to act as a contingency resource. There are numerous types of ancillary services that can be provided, several ancillary services are described below and how batteries can participate as such.

Frequency Regulation: Frequency regulation ensures the balance of supply and demand of electricity over timeframes of seconds to minutes. This automatic change to match supply and demand is required to maintain the frequency within the statutory and operational limits, typically 60 Hz or 50 Hz depending on region. As supply is mismatched with demand, batteries can absorb power. Vice versa, when demand is higher than supply, batteries can dispatch into the grid and provide more supply.
Voltage Control: Voltage control is a critical feature of the electrical grid. Controlling voltages enables the efficient transmission of power while considering device limitations. The voltage that is provided to your house is at 120 and 240 volts in the US and Canada. The equipment and tools we have that run on electricity require a specific voltage to operate correctly or else major damage will occur. On a grid scale, the same issue exists. Certain elements of the electrical grid are rated for certain voltages, batteries can help to ensure that the proper voltage is being used. Batteries provide voltage control by maintaining a consistent voltage output.
Spinning Reserves: Spinning reserves are those that are synchronized to the frequency of the system and can therefore provide electricity upon being called. Spinning reserve is used for protection against contingency events such as the failure of a generator or downing of a transmission line. These resources can be brought online quickly to replace the loss of a resource and allow other resources to come online to meet the demand. Batteries can respond instantly to this need and can provide spinning reserve through synthetic inertia.
Black Start: Black start refers to a situation in which a resource has the ability to restart parts of the power system to recover from a blackout. This essentially means that power stations are started individually and eventually reconnected to become an interconnected system again. Given this importance, a resource having black start capability is incredibly important for system reliability and resilience. Batteries can provide black start capability through provision of backup power to infrastructure on the grid such as generation resources, transmission lines, and distribution networks.
Reactive Power: Reactive power is the power that flows back from a destination toward the grid in an alternating current scenario. Our grid is currently alternating current, batteries actually provide direct current. While on their own, this means that batteries cannot provide reactive power, coupled with an inverter that transfers the current to alternating current, batteries can provide reactive power
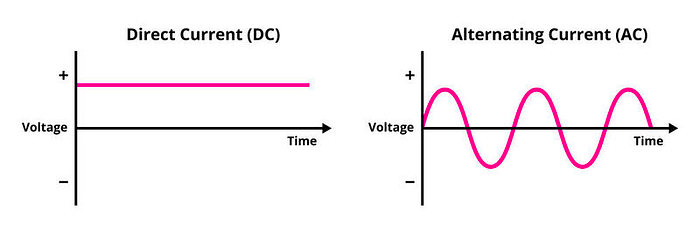
The above mentioned ancillary services do not represent the full options available for BESS assets to earn revenue from but provide a high level overview of some key ones. In summary of ancillary revenues, batteries can provide these ancillary services to the grid, and in return, can earn revenue and are a component of the value stack.
4 — Tolling
A tolling agreement is an agreement in which one party, the toller, provides fuel and offtakes electricity from a generation asset that is dispatched by the generations asset operator based on the toller’s specifications. Importantly, the generation asset owner retains control of the battery and is responsible for maintaining it. Under the context of batteries, a tolling agreement refers to a customer paying a toll to use the battery’s capacity and services.
BESS assets have access to a variety of revenue steams and to unlock the true value. Operators, developers, investors, and other parties must look toward value stacking to create the most compelling opportunity. The best option for these BESS assets is that of contracted revenue, of capacity payments, tolling agreements, and any other assured sources of revenue backed by strong contracts. These contracted streams are more financeable than merchant market revenue streams and thus make capital raising for these projects easier.
Operational Parameters
As discussed, batteries have some operational quirks based on the technology that impact the economics. The two quirks of batteries that I would like to briefly touch on are degradation, lifespan, and efficiency and how these two impact the economics of a BESS asset.
Degradation
As batteries age and get used, the storage capacity decreases and the internal resistance increases. These changes are due to a range of degradation mechanisms that impact the battery that occurs simultaneously or cause future degradation mechanisms. Certain usage traits of the battery can also impact degradation with a report from Fitch Ratings highlighting that a high proportion of arbitrage in the value stack for batteries could cause further degradation and impact the lifespan of the asset. There are three main external stress factors that impact batteries, degradation, temperature, state of charge, and load profile.
“In general, temperature is the most significant stress factor, where deviations from the typical 25 °C can lead to accelerated failure. Higher SoC operation accelerates degradation, due to the relationship between the electrode potentials and the rate of parasitic side reactions, while higher current operation increases the likelihood of failure, due to mechanical stresses developing in the battery during cycling, but also promotion of lithium plating during charge.”
-Edge et al., 2021
In short, the degradation of a battery reduces the effective capacity. As such, depending on contract terms, batteries may be required to be oversized to ensure that contractual terms for delivery of energy can be met. To offset the impact of degradation, batteries can implement augmentation strategies to ensure that degradation limits the reduction in capacity. This is touched on further in the operating costs section.

Lifespan
The lifespan of BESS assets depend on a variety of factors such as operations & maintenance methodology, augmentation strategy, and ambient conditions such as temperature, humidity and weather. On average, BESS assets (lithium ion) have a life of 20 years. Again, highly dependent on several key assumptions on operating profile.
Efficiency
Another key operational parameter that impacts the economics of a BESS asset is that of a battery’s efficiency. Battery efficiency is defined as the ratio of electrical energy produced by the BESS asset relative to the amount of chemical energy it stores. It measures how effectively a battery can convert stored chemical energy into useable electrical energy. Formulaically put:
Efficiency = Output Energy / Input Energy
Efficiency of a battery essentially translates to the fact that more energy is required to charge the BESS asset than energy can be dispatched to the grid. This fact is especially important to energy arbitrage revenue strategies as it impacts what your charge price and discharge price must be in order to actually generate cash flow. Essentially, the spread between your discharge price and charge price must account for the increased costs of charging relative to discharging. For reference, lithium-ion batteries are ~95% efficient. Some reasons for batteries not being perfectly efficient include energy loss due to internal resistance, self-discharge, environmental factors, and chemical reaction failure.
Capital Costs
The largest cost incurred in the lifecycle of a BESS asset is that of the upfront capital cost or capital expenditure. This is the cost to construct the BESS asset. Given the nascent nature of utility-scale batteries, there is considerable wide ranging estimates on the true capital cost of the technology. In addition, the fact that the capital costs can be dependent on desired configuration, operating profile, and ambient conditions, means that significant uncertainty exists on identifying the true cost. With that in mind, the figures I have below are indicative of an amalgamation of estimates for the current price of a BESS asset as well as the National Renewable Energy Laboratory’s estimate for future capital costs.

The above image is very good at establishing the playing field as of 2022 for the current cost of a 4-hour battery in $/kWh. While estimates vary depending on the source, the likely range appears to fall between ~$300/kWh to ~$550/kWh. The expression of capital cost is on a $/kilowatt-hour basis to account for the pricing of the system accounting for the size (kW) and duration (hours) as both elements are important to capture the true cost of a battery system.
These figures are set to decline as the technology is increasingly adopted and becomes more well deployed and produced, benefitting from the learning curve effect. Below you can find two images on the estimated future cost of battery assets as well as actual decline in cost of solar modules. The reference to solar modules is important to understand and validate the expected decline in battery costs as an illustration of the learning curve.
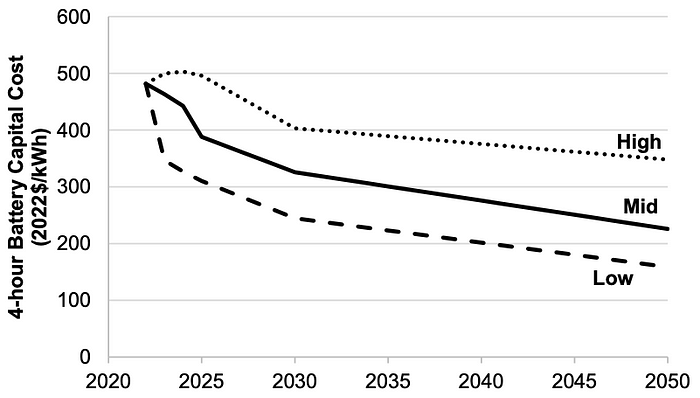
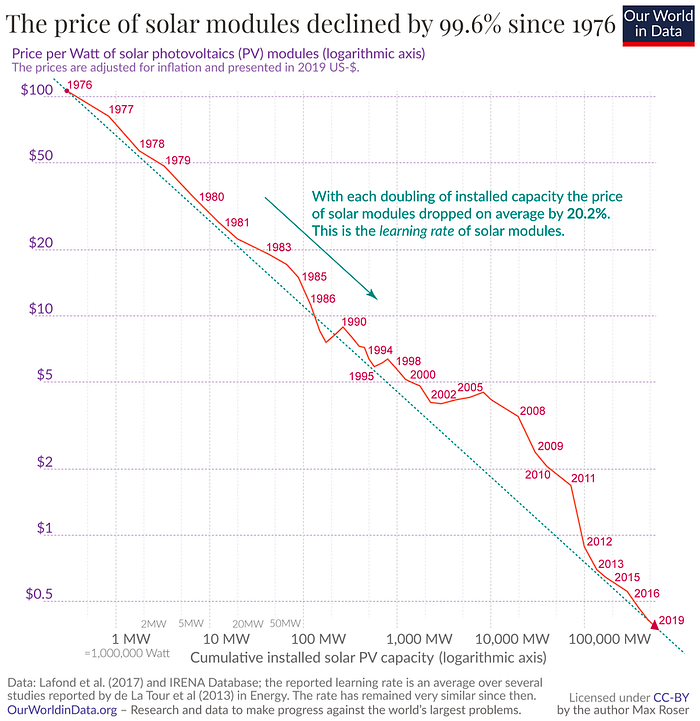
As an aside on learning curves, learning curves are essentially the relationship between units produced and costs to produce them. It describes how the production cost decreases as production quantity increases. In the case of solar energy, learning curves apply to the installation cost of solar systems and the $/W of electricity capacity installed. As more solar systems are installed and the technology advances, the installation and operating costs decrease over time. This is due to increased efficiency, economies of scale, investment in research and development, and improved manufacturing processes, among other factors. Now back to batteries.
The actual battery is composed of several elements that each have a cost. When examining the breakdown of materials costs, the electrode materials (anode, cathode, electrolyte) are approximately 50% of the total costs. Components include:
- Anode — The electrode where oxidation occurs during the discharge of a battery.
- Cathode — The electrode where reduction occurs during the discharge of a battery.
- Electrolyte — A substance that allows the flow of ions between electrodes in a battery, facilitating the chemical reactions that produce electricity.
- Separator — A material that physically separates the anode and cathode in a battery while allowing for the flow of ions.
- Other Materials — Materials used in battery manufacturing.
- Labour — The cost of the human resources involved in the production of batteries.
- Overhead — The cost of overhead expenses associated with battery production, such as rent, utilities, and maintenance.
- Profit Margin — The amount of profit added to the cost of producing and selling batteries.
- Rest — Other factors that may impact battery production and cost
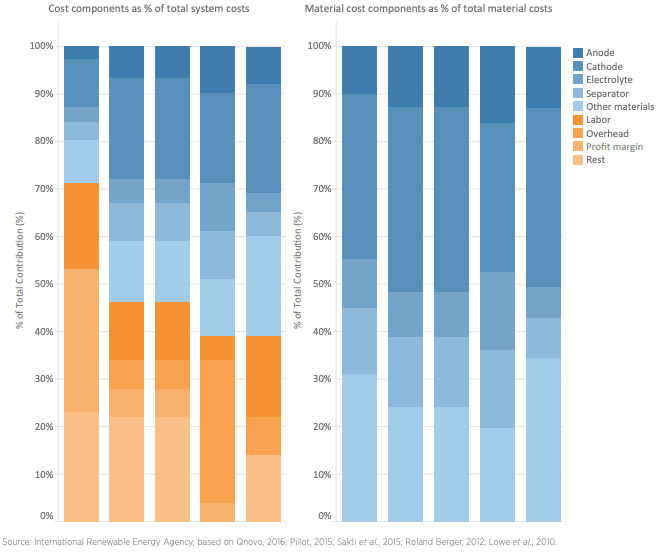
The capital cost of li-ion batteries have declined on average in recent years and are expected to decline into the future. This decline is led by increased demand for electric vehicles but also stationary batteries. The costs of utility-scale batteries also must include components such as balance of system, power conversion system, energy management system, and EPC costs.
Operating Costs
The operating costs for utility-scale BESS (Battery Energy Storage Systems) are generally low, as they do not require fuel or extensive maintenance. However, they do require periodic replacement of batteries (which constitute the major cost of the unit) and inverters. The frequency of battery replacement depends on the type of battery used, the depth of discharge, and the operating conditions. To minimize replacement costs, careful control of operating conditions is critical. In addition, a system for recycling and disposal of used batteries must be in place to ensure compliance with environmental regulations.
Maintenance of a utility-scale BESS typically involves regular checks on the system’s components and condition, and cleaning of the applicable parts. The maintenance cost of a BESS facility is generally low relative to the initial investment and the revenue the system can generate. However, as the system ages, maintenance requirements and costs will likely increase. The system’s performance will also decline over time as the batteries lose capacity, which will need to be factored in when considering maintenance requirements. Overall, the operating and maintenance costs for utility-scale BESS are relatively low compared to traditional power generation assets and can provide significant value to the grid.
The cost of battery augmentation depends on various factors, such as the type of battery, its size, and the level of augmentation required. Typically, lithium-ion batteries are the most expensive to augment due to their complex structure and high energy density. Additionally, larger batteries require more materials and complex manufacturing processes, making them more expensive to augment. The level of augmentation required also plays a significant role in determining the cost, as minor improvements may be achieved through simple adjustments, whereas significant increases in performance require more extensive modifications. However, advancements in technology and economies of scale are driving down the costs of battery augmentation, making it more accessible to a wider range of industries and applications.
Expressed as a percentage of the LCOS of a battery, the O&M costs are in the range of 16% to 24% for current installations. Transmission and distribution scale applications are in the range of 22% to 24% and utility-scale behind-the-metre applications sited with solar usually are in the range of 16%-19%.
Disposal Costs
After the battery comes to the end of its useful life, there are two broad pathways a battery can follow; reuse or disposal. While there are many exciting developments in the world of battery recycling such as Redwood Materials and Ascent Elements in the US, the vast majority of batteries are disposed of. Given the uncertainty around the disposal of BESS assets in the future, the disposal cost may actually be an inflow of cash if recycling companies are willing to pay for this material. Given the uncertainty, it is difficult to say how this item is best modelled.
Conclusion
Only by combining the revenue streams available, operating characteristics, capital costs, operating costs, and disposal costs can we understand the true economics of a BESS asset. The calculation of returns for a BESS asset is highly dependent on site specific information and details about the required operating characteristics for the technology. A highly simplistic view of the cash flow profile for a BESS asset may be as shown below. As you can see, the construction of the asset is where the initial outlay of cash flow is, followed by the 20 year lifespan of the asset in which it incurs operating costs and earns revenue.

BESS assets play a vital role in the energy transition towards a greener and more sustainable future. The intermittent nature of renewable energy sources such as solar and wind makes the integration of these sources into the power grid challenging. BESS can store excess energy generated during peak hours and supply it when renewable energy sources are not producing, allowing for more efficient power generation. Additionally, BESS allows for energy to be stored locally, making it more accessible and reliable for communities. With the increasing demand for clean and sustainable energy, the importance of BESS in the energy transition cannot be underestimated.
Sources
“Ancillary Services.” Greeningthegrid.org, 2013, greeningthegrid.org/integration-in-depth/ancillary-services#:~:text=Introduction,after a power system event. Accessed 14 Sept. 2023.
“Ancillary Services · Energy KnowledgeBase.” Energyknowledgebase.com, 2018, energyknowledgebase.com/topics/ancillary-services.asp. Accessed 14 Sept. 2023.
“Battery Lifetime, Efficiency and Care.” Wind & Sun, 2023, www.windandsun.co.uk/blogs/articles/battery-lifetime-efficiency-and-care#:~:text=Battery Efficiency,-No battery is&text=Its efficiency is a measure,80kWh can be taken out. Accessed 14 Sept. 2023.
“Battery Storage Value Stacking | Enel North America.” Enelnorthamerica.com, 7 July 2023, www.enelnorthamerica.com/insights/blogs/battery-storage-value-stacking. Accessed 13 Sept. 2023.
“Black Start.” Nrel.gov, 2023, www.nrel.gov/grid/black-start.html#:~:text=Black start is the ability,form an interconnected system again. Accessed 14 Sept. 2023.
Edge, Jacqueline, et al. “Lithium Ion Battery Degradation: What You Need to Know.” Physical Chemistry Chemical Physics, vol. 23, no. 14, 1 Jan. 2021, pp. 8200–8221, pubs.rsc.org/en/content/articlehtml/2021/cp/d1cp00359c, https://doi.org/10.1039/d1cp00359c. Accessed 14 Sept. 2023.
Energy storage — IEA. “Energy Storage — IEA.” IEA, 2023, www.iea.org/energy-system/electricity/grid-scale-storage. Accessed 13 Sept. 2023.
“Financing Energy Storage Projects: Assessing Risks | Norton Rose Fulbright.” Projectfinance.law, 2017, www.projectfinance.law/publications/2017/june/financing-energy-storage-projects-assessing-risks. Accessed 13 Sept. 2023.
Henderson, Sarah. “Understanding Battery Energy Storage Systems (BESS) and Power Arbitrage by SPE Electrical Ltd.” Power and Cables, 15 June 2021, www.powerandcables.com/understanding-battery-energy-storage-systems-bess-and-power-arbitrage-by-spe-electrical-ltd/. Accessed 13 Sept. 2023.
“How Do Batteries Work?” Northwestern.edu, 2023, www.qrg.northwestern.edu/projects/vss/docs/power/2-how-do-batteries-work.html. Accessed 13 Sept. 2023.
James, Luke. “What’s the Difference between AC and DC Power?” Power-And-Beyond.com, Power & Beyond, 19 Mar. 2020, www.power-and-beyond.com/whats-the-difference-between-ac-and-dc-power-a-0c5c48e598b5e1266e6cebc5731227c2/. Accessed 14 Sept. 2023.
Kavya Balaraman. “Battery Storage Systems Could Face Rapid Asset Degradation, Especially with Arbitrage: Fitch.” Utility Dive, 18 July 2023, www.utilitydive.com/news/battery-storage-systems-rapid-asset-degradation-arbitrage-fitch-ratings/688275/#:~:text=from your inbox.-,Battery storage systems could face rapid asset degradation%2C especially with,increase%2C according to the analysis. Accessed 14 Sept. 2023. “LinkedIn.” Linkedin.com, 2023, www.linkedin.com/pulse/revenue-streams-bess-amy-jo-miller/. Accessed 13 Sept. 2023.
mischa. “How a Battery Works.” Curious, Nova, 24 Feb. 2016, www.science.org.au/curious/technology-future/batteries. Accessed 13 Sept. 2023.
Noussan, Michel. “Economics of Electricity Battery Storage.” ResearchGate, unknown, 30 May 2022, www.researchgate.net/publication/360937787_Economics_of_Electricity_Battery_Storage. Accessed 13 Sept. 2023.
“Spinning Reserve · Energy KnowledgeBase.” Energyknowledgebase.com, 2018, energyknowledgebase.com/topics/spinning-reserves.asp. Accessed 14 Sept. 2023.
Sriram Emani. “Spinning Reserve Displacement: Using Batteries for a More Efficient and Cleaner Way to Back up Power.” Renewable Energy World, 23 June 2020, www.renewableenergyworld.com/storage/spinning-reserve-displacement-using-batteries-for-a-more-efficient-and-cleaner-way-to-back-up-power/#gref. Accessed 14 Sept. 2023. “Tolling Agreement | Practical Law.” Practical Law, 2023, uk.practicallaw.thomsonreuters.com/8–623–0265?transitionType=Default&contextData=(sc.Default)&firstPage=true#:~:text=As used for electricity%2C an,agreement or toll processing agreement. Accessed 14 Sept. 2023.
“What Types of Batteries Are Used in Battery Energy Storage Systems? — WattLogic.” WattLogic, 20 Feb. 2021, wattlogic.com/blog/types-of-battery-energy-storage-systems/. Accessed 13 Sept. 2023.